High current PCB design
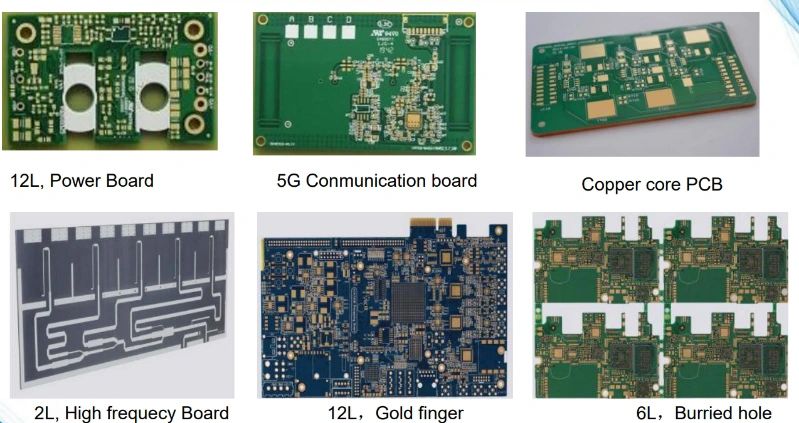
High current PCBs are printed circuit boards designed to safely distribute and control large amounts of electrical current within an electronic system. Where a typical PCB handles current levels up to 5-10A, high current PCBs can manage currents from 50-100A up to 1000A or more flowing through conductor traces. They enable complex power electronics and high power devices to be efficiently packaged.
High current PCB design demands managing sizable thermal loads and IR power losses. It requires the use of robust board and layout techniques to create reliable, long-lasting circuitry. When done effectively, high current PCBs unlock substantial applications requiring compact, customized power distribution solutions.
Design Considerations
To translate a high current system concept into a manufacturable PCB assembly, designers must make considerations in a number of key areas:
Power Supply
Selecting Appropriate voltage/current rated power modules
Planning stable power input conditioning
Allowing sufficient cooling of converters
Conductor Sizing
Using appropriately sized copper weights for current levels
Minimizing impedances for lowest losses
Allowing for temperature rise at load
Trace Routing
Utilizing shortest path between terminals
Minimizing 90° bend angles
Sufficient spacing between neighboring traces
Thermal Management
Calculating potential trace temperature rise
Incorporating thermal reliefs and thermal breaks
Allowing for sufficient conductor heat sinking
Safety and Protection
Preventing access to live terminals and bus nodes
Incorporating current monitoring instrumentation
Adding fusing/protection elements
High Current PCB Materials
Choosing suitable PCB materials is essential to handle the demands of high current applications:
Substrates
FR-4 Glass Reinforced Epoxy - Cost effective; usable to ~50A.
Polyimide - Excellent thermal performance for high temp flexibility applications.
Aluminum - Direct bond substrate for highest thermal capacitance.
Ceramic - High dielectric insulation properties. Withstands 1000+A.
Copper Weights
Higher copper thicknesses used to minimize resistances and supply current capacity
1oz (35um) copper - Only usable for very low current applications.
2oz (70um) copper - Marginal current handling; minimal heat spreading.
3oz+ (105um+) copper - Preferred for high current PCBs carrying 50A+.
Coatings/Finishes
Immersion Tin - Most common final finish providing excellent solderability.
ENIG - Withstands high current flow but thick gold inhibits heat transfer.
HASL - Lead-tin plating builds copper thickness; efficient cooling.
Layout Techniques
High current PCB layouts utilize various routing arrangements to effectively distribute large currents:
Layout StyleDescriptionStar DistributionSeparate paths radiating from central power pointDaisy ChainingSerial conductor loops strung between terminalsPower PlanesSolid copper fill areas for spreading current in 2D areaBus BarLinear row of joined pads forming high current backboneHybridMixing different distribution patterns on same board
Common high current layout practices:
Minimize overall trace lengths using optimal component placement
Use impedance-matched symmetrical trace splitting from nodes
Connect multiple vias in parallel to share current loads
Expand contact areas to lower interface resistances
Representative Applications
Some of the key application areas utilizing high current PCB technology include:
LED Lighting
Drive hundreds of watts into massive LED arrays
Manage heat flux from high power solid state lighting
Power Distribution
Replace bulky wiring harnesses and buswork
Route battery/converter outputs
Motor Drives
Switch high DC currents in electric vehicle systems
Distribute three phase outputs to control industrial motors
Power Conversion
Connect high current ports in VFDs and AC/DC converters
Provide precision current sensing and measurement
Battery Management Systems
Route currents in electric vehicle charging systems
Monitor cell-level voltages/currents in battery packs
Thermal Considerations
The passage of high currents through printed circuitry results in resistive power losses and temperature rises which must be dissipated through adequate cooling provisions:
Sources of Heat
I<sup>2</sup>R losses - Joule heating from currents through metal resistances
Magnetic losses - Hysteresis and eddy currents in components
Dielectric losses - Leakage current flows across insulating substrates
Impact of Temperature
Thermal expansion and mechanical stresses
Decreased electrical/thermal conductivity
Accelerated chemical degradation mechanisms
Cooling Methods
Conduction cooling - Heat sink attachments to spread heat
Convection cooling - Fans blowing air over circuits
Liquid cooling - Cold plates, embedded heat pipes
Adequate thermal design ensures conductors remain within safe operating temperatures and prevents thermomechanical damage.
Design Verification
Prior to full scale production, high current PCB designs require rigorous verification:
Simulation
Predict current density distribution and resistive voltage drops
Estimate maximum temperature rises under load
Prototyping
Validate assembly procedures and workmanship quality
Confirm proper interconnection functionality
Testing
Monitor heat profiles using thermal imaging cameras
Quantify voltage drops across transmission paths
Assess insulation resistance and dielectric strength
Verify operation during long duration power cycling
Iterative testing enables incremental optimization of layouts, materials selections and fabrication approaches.
Future Outlook
Emergent wide bandgap semiconductor technologies including SiC and GaN will enable higher frequency, higher efficiency power electronics. This drives demands for improved high current PCB solutions. Continued materials advancements and modeling sophistication will facilitate reliably routing thousands of amps through printed circuits.
Summary
With careful planning and verification, high current PCB technology provides a customizable framework for efficiently distributing and controlling large electric currents. When layout considerations are married with appropriate materials selections, thermal management provisions and design testing, printed circuits can manage currents exceeding 1000A in advanced power electronic systems.
Frequently Asked Questions
What design tools are used?
High current PCB layouts leverage specialized modelling features within major EDA software platforms to accurately represent thermal profiles and specify constrained topologies. Advanced verification occurs through linked electrical, thermal and mechanical modelling tools.
What fabrication challenges exist?
Fabricating high current PCBs requires advanced processes to meet minimum copper thickness requirements, ensure interlaminar bond integrity, minimize burring, and properly plate/coat conductors. Careful process control prevents defects which lead to hotspots.
Is post fabrication machining used?
For extremely high current applications, additional machining like edge milling or copper shaping may be applied after standard PCB fabrication to further increase conduction area and customize current terminals.
How are components attached?
Attaching discrete components to high current pads demands very robust, low resistance soldering or bonding approaches. Reflow soldering may be supplemented with selective hand soldering. Direct bonded copper and active solders provide ideal interconnections.
What inspection modalities are used?
Inspection requires verifying copper integrity across the entire high current conduction path. X-ray imaging modalities allow inspection through overlaying structures to completely examine buried features. Thermal profiling under load highlights defects before product shipment.